
Advancing Planetary Surface Observation Techniques
Exploring Planetary Surfaces: Bridging Terrestrial and Extraterrestrial Environments
Amid creeping dunes, sage brush, and dry grass, a large metal framework spreads appendages over a sandy plain like an aluminum insect. On antenna-like poles, instruments twitch and spin as they sense the air.
This scene, otherworldly yet altogether terrestrial, played out in June 2022 at Bruneau Dunes State Park in south central Idaho. As part of a NASA-supported workshop, a group of a few dozen planetary scientists, terrestrial geologists, and engineers experimented with the insect’s instruments and clambered over the dunes to investigate how to explore other worlds. The metal framework was a mock-up of a planetary lander concept under development by the Jet Propulsion Laboratory, and the dune field provided a natural laboratory to study how best to observe surface-atmosphere interactions accurately on a world like Mars.
Interactions between planetary surfaces and their atmospheres play crucial but underappreciated roles in shaping worlds across our solar system, and we can study these interactions by connecting terrestrial field experiments with extraterrestrial observations. After all, the same kinds of wind stresses that drive Bruneau’s dunes also sculpt the sand seas of Saturn’s moon Titan, carving the moon’s climatic history into its dunes. And dust-lofting processes similar to those on Earth likely also conjure dust storms on Mars, perhaps helping explain that planet’s extreme aridity.

By studying terrestrial surface-atmosphere interactions, scientists hope to understand how the forces at work here also affect distant worlds, where their influences may be more profound but also more cryptic. Recent planetary missions, data sets, and experiments have provided insights, but key questions remain. Fortunately, new and upcoming instruments, data collection approaches, and planetary missions that will help answer these questions lie on the horizon.
Surface-atmosphere interactions influence many worlds, but Earth, Mars, and Titan share compelling similarities and contrasts, so we focus here on this trio of tantalizing terrenes.
Dunes and Don’ts
Dunes and their smaller cousins, ripples, are observed across the solar system, from the bottom of Earth’s oceans to the frigid surface of Pluto.
Dunes and their smaller cousins, ripples, are observed across the solar system, from the bottom of Earth’s oceans to the frigid surface of Pluto—anywhere a moving fluid passes over sediment grains. Winds can mobilize grains, but only if they are fast enough to overcome the gravity that holds grains down—especially larger grains—and the interparticle forces that hold smaller grains together. With these forces at play, there is, on any world, a range of grain sizes that are easiest for winds to move. Grains in that size range are traditionally called “sand.”
Sand grains move in several ways, including by saltating (hopping), creeping (rolling), and reptating (the splashing of grains caused by impacts of other high-velocity grains). Once mobilized, sand grains can collect in dunes and ripples with a variety of shapes that reflect localized and regional wind patterns. On Earth, dunes are typically confined to desert regions where sand is unlikely to be trapped or transported away by water. On Mars, dune fields are widespread—time-lapse imagery collected from Mars orbit shows dunes as tall as 100 meters in motion all across the planet. These images also provide some of the only direct information we have about Martian seasonal wind patterns, effectively serving as regional-scale anemometers.
The discovery of dunes on Saturn’s icy moon Titan, meanwhile, has prompted intriguing speculations about connections between climate and sand grain formation. Nearly 20% of Titan’s surface is covered in dunes that wrap around the equator; the widths and spacing of these dunes are up to 3 kilometers, and their shapes resemble terrestrial linear dunes.

Unlike the mineral sands of Earth’s and Mars’s dunes, though, Titan’s dune sands are composed of hydrocarbons. Photochemical reactions in Titan’s methane-rich, nitrogen-dominated atmosphere produce a continual rain of macromolecular aerosols that are probably the source of the moon’s sands, but how micrometer-sized aerosols become 100+-micrometer-diameter sand grains is unclear. One model suggests that the aerosols gather in polar lakes of liquid natural gas, where turbulence clumps them together. When these lakes dry up seasonally, the clumps are left behind to be distributed by wind around the rest of the moon. This same clumping process, called “flocculation,” forms sand in coastal lake beds in Morocco, for example. Seasons on Titan last 7 Earth years, but a longer 60,000-year cycle associated with Saturn’s orbital evolution (analogous to Milankovitch cycles on Earth) likely contributes to more extensive, long-term evaporation cycles.
Despite the rich insights provided by previous work on dune dynamics on both Mars and Titan, many loose ends remain.
Despite the rich insights provided by previous work on dune dynamics on both Mars and Titan, many loose ends remain. For example, recent lab experiments conducted under very low atmospheric pressures similar to those on Mars revealed an unexpected fluid-particle interaction that seems to substantially lower the threshold wind speed needed to mobilize sand on Mars. This effect may help explain how Mars’s whisper-thin atmosphere can whip its global sand seas into motion, but confirming whether the effect actually applies on Mars requires in situ, high-frequency monitoring of surface conditions.
Titan-tuned trials with analogue hydrocarbon sand grains suggest the moon’s dunes may experience substantial static charging. This static charge might render them aerodynamically resistant to Titan’s gentle, but continuous, eastward equatorial winds. Perhaps only the unusually vigorous westward winds that accompany Titan’s seasonal storms are capable of moving the dunes.
Dust in the Wind
Sand isn’t the only kind of sediment that interacts with wind. Whereas sand grains usually hop along the surface, dust particles—just micrometers to tens of micrometers in diameter—can really take wing. Indeed, a key feature of dust is that it can remain suspended in the skies over long distances. Dust from the Sahara regularly travels thousands of kilometers over the Atlantic Ocean to fertilize the Amazon rain forest, for example. And once in the air, dust can affect atmospheric and climatic conditions on global scales.
Unlike on Earth, carbon dioxide in Mars’s wan atmosphere provides little warming. Instead, perpetually suspended dust absorbs sunlight, increasing atmospheric temperatures by tens of degrees Celsius. This warming weakens the atmospheric cold trap that keeps water near the surface and helps generate more dust storms that maintain the warming and lift water high in the atmosphere, thus accelerating its loss to space. Data from NASA’s Mars Atmosphere and Volatile Evolution (MAVEN) mission suggest this dust-boosted escape may help explain why Mars is so dry today.
Cassini’s infrared images suggested regional dust storms rose as high as 10 kilometers over Titan’s surface and lasted at least 11 hours.
Titan is also a dusty world. NASA’s Cassini mission, which spent 2004 to 2017 exploring the Saturn system, imaged regional dust storms spilling across Titan’s equatorial dunes. Cassini’s infrared images suggested these storms rose as high as 10 kilometers over the surface and lasted at least 11 hours, analogous to, but more monstrous than, haboobs on Earth.
Despite dust’s broad reach, the exact mechanisms that mobilize it remain obscure. Although significantly lighter than sand grains, dust grains can be more difficult to move—their smaller sizes imply not just smaller masses but also smaller drag surfaces and stronger interparticle cohesion.
Theoretical estimates considering only fluid drag and interparticle forces suggest that near-surface winds on Mars must exceed 40 meters per second (90 miles per hour) to lift 1-micrometer dust grains. But such wind speeds are very rarely observed. Titan’s thicker atmosphere (more than 4 times Earth’s atmospheric density) and lower gravity (one seventh of Earth’s) may imply that dust there is easier to lift. Even so, wind tunnel experiments suggest that 5-micrometer-wide dust on Titan requires winds of 1 meter per second to move, yet surface winds on the moon rarely exceed a few centimeters per second. So either dust rarely moves on Titan, or like on Mars, something more than fluid drag is at work.

In fact, sand likely helps to mobilize dust. Because sand is easier to set in motion than dust is, as wind gusts pick up speed, sand moves first. As the sand saltates, each grain can accumulate substantial momentum, and when sand grains impact the surface, this momentum helps launch dust grains. This cascading effect likely reduces the threshold wind speed for mobilizing dust on Mars, probably to within observed wind speeds. The InSight (Interior Exploration using Seismic Investigations, Geodesy and Heat Transport) mission on Mars even leveraged this effect to prolong its own life: InSight ladled sand onto its increasingly dusty solar panels, resulting in a power boost as the blown sand grains helped clean the panels.
Water, Water Everywhere, Nor Any Drop to Drink
Turbulent winds near planetary surfaces do more than move sand and dust—they also transport atmospheric gases that can evaporate, condense, and influence planets in dramatic and sometimes unexpected ways. From the deep mantle to the troposphere, Earth is sodden with water, which affects almost every aspect of our planet’s climatic, geological, and biological systems.
On Mars, surface conditions preclude the presence of large amounts of liquid water, but Mars has water ice in abundance. In situ and orbital measurements of Mars have revealed an intricate water cycle akin to Earth’s. On Titan, where the surface temperature is about −180°C, water similarly exists only as ice, but methane and ethane fall as rain and fill large surface reservoirs. Although less well characterized than Earth’s or Mars’s, Titan’s “hydrologic” cycle (with methane and ethane playing the role of water) seems intimately woven into that world’s climate and geology.
Even such a thin layer of frost can drive weathering of Mars’s surface, which might explain ubiquitous spectral signatures of hydration observed from orbit.
In situ measurements on Mars suggest that in the deep cold of the Martian night, water vapor condenses out across the rocky surface as a frost layer, probably only a few micrometers thick. Even such a thin layer of frost can drive weathering of the surface, though, which might explain ubiquitous spectral signatures of hydration observed from orbit.
Interactions between atmospheric water vapor and Mars’s salty surface might also lead to deliquescence. That is, solid mineral salt deposits may absorb water vapor, forming hydrated solid minerals or even very salt rich brines that could trigger observed seasonal mass-wasting movements on slopes. Of course, water is a requirement for life as we know it, so scientists are keen to understand these surface-atmosphere interactions on Mars.
The origins of Titan’s abundant methane and ethane are unclear. Various atmospheric escape and destruction processes are thought to remove these volatiles over geological timescales, but they still dominate Titan’s hydrologic cycle, which involves global transport, infrequent but violent convective storms, and erosion of the moon’s icy surface.
The only available in situ data set from Titan comes from the Huygens spacecraft, which collected measurements for several hours as it descended via parachute through Titan’s atmosphere and landed near the equator in January 2005. Among other data, Huygens recorded a profile of atmospheric pressure, temperature, and composition during its descent.
These data revealed a methane relative humidity well below the value thought to be required to trigger a convective hydrocarbon rainstorm, in spite of the fact that large methane and ethane storms were observed by the Cassini mission. Meteorological modeling of Titan suggests that global atmospheric dynamics transport methane away from the equator toward the poles, rendering the equator arid. However, Huygens observed a surface wetted with liquid methane, perhaps indicating that subsurface reservoirs resupply the surface.
More Information, Please
The above observations highlight the complexities of sediment transport and volatile exchange near planetary surfaces.
Understanding Mars’s water cycle, including the significance of deliquescence, for example, will require additional experimentation and modeling, leavened with more refined in situ measurements. The degree to which deliquescence occurs depends on the amounts of salts and water vapor available, transport of water through the rocky surface, and temperature, which strongly affects the kinetics of deliquescence and efflorescence (the transition back to a solid salt via dehydration). Near-surface atmospheric profiling would help scientists quantify the availability, diurnal variations, and surface fluxes of water vapor.
On Titan, such profiling collected throughout a Titan day (16 Earth days) would help clarify surface-atmosphere exchanges of volatiles there as well. Studying subsurface porosity and methane storage would also provide insights into Titan’s meteorology and, ultimately, the origins of its volatiles.

Missions, instrumentation, and techniques already available and in development may soon provide new capabilities to understand surface-atmosphere interactions that so profoundly shape worlds throughout the solar system. Which leads us back to Bruneau Dunes State Park here on Earth.
Participants in NASA’s “Optimizing Planetary In Situ Surface-Atmosphere Interaction Investigations” workshop circled the lander mock-up as a fitful breeze drove sand streamers around their ankles. The aluminum framework was outfitted with wind sensors, temperature gauges, and other atmospheric instruments, and the group discussed which ones could best assess saltation in Bruneau’s arid environment.
Many of the most important surface-atmosphere interactions are intermittent, stochastic, and unpredictable. Continuous data collection could capture these interactions.
The conversation then turned to the challenges of balancing the limited bandwidth for beaming mission data back to Earth from another world and other mission constraints against the need for more and higher-fidelity data. The vigorous, but sporadic, saltation of sand underscored the difficulty: Many of the most important surface-atmosphere interactions are intermittent, stochastic, and unpredictable. Continuous data collection, expensive in terms of bandwidth, could capture these interactions, whereas intermittent data collection risks missing many or all of them.
Brute Force, Playing the Odds, or Artificial Intelligence and Drones
In terrestrial studies, the solution to such challenges is often to collect data frequently and for long periods. For example, a U.S. Geological Survey–led field study of ripple migration on the Grand Falls Dune Field in Arizona collected images every 10 minutes for 128 days. Comparing this sustained, high-cadence imagery to concurrently collected meteorological data allowed researchers to relate the migration rates of the ripples to wind speeds. However, this experiment produced about 11 GB of data and had an average image data production bandwidth that was almost 10 times that of Insight on Mars.
Previous planetary missions have sidestepped bandwidth issues by limiting high-frequency sampling to only times when the phenomena of interest were expected to occur. The camera team for the Mars Spirit rover scheduled several high-cadence (3-second sampling) imaging sequences around local midday (“mid-sol”) to image dust devils, which are believed to be a key contributor to the planet’s dust budget. This surveying netted sightings of 533 devils over about 10 hours of observing. However, a similar campaign by Insight saw no dust devils during about 111 hours of observing.
Instead of trying to time expensive observations cleverly, it’s possible that missions could be designed so that certain environmental conditions trigger observations. Machine learning could help determine the threshold conditions that would most effectively allow dust devil encounters to be documented, for example. Dust devils are relatively small-scale phenomena embedded in larger-scale atmospheric structures (1 kilometer or more across) that drive increases in wind speed. Gaussian process techniques incorporating complex noise models and applied in machine learning algorithms could distinguish these atmospheric structures from random turbulence in meteorological data.
Furthermore, the onset of threshold environmental conditions could be set to trigger several instruments at once, not just cameras. By luck, the Perseverance rover on Mars recently detected a direct hit by a dust devil using many instruments simultaneously, including cameras, wind sensors, and even its microphone, which registered the impacts of individual grains. This data set may allow scientists to estimate the dust flux from a dust devil directly and could inspire future mission designs.
Novel platforms promise fresh insights. Flying drones, for example, may become a preferred means to probe near-surface atmospheres on other worlds.
Novel platforms also promise fresh insights. Flying drones, for example, may become a preferred means to probe near-surface atmospheres on other worlds. As a technology demonstration, the Ingenuity helicopter operating on Mars carries no scientific instruments, but it has already shown the potential of air-based exploration for studying the planet’s surface and atmosphere over broader areas than is possible with landers or rovers. The Dragonfly rotorcraft, slated to arrive on Titan in the mid-2030s, will have more capabilities than Ingenuity. Dragonfly will carry meteorological instruments, high-resolution cameras, and various spectrometers, providing unprecedented insights into the surface-atmosphere interactions and more on that distant world.
Profiling near-surface volatile atmospheric compounds with drones will help constrain surface-atmosphere fluxes, for instance, and profiling near-surface wind speeds can help determine threshold wind stresses required to mobilize sand and dust. Indeed, ongoing research has suggested that even though Ingenuity carries no wind sensors, data collected on its attitude (i.e., the helicopter’s orientation with respect to the horizon) carry information about the wind profile.
All these possibilities and more fired the imaginations of the scientists and engineers as they piled into vehicles and drove back from the field at the end of the excursion in the Bruneau dunes. Key scientific and technical questions about studying surface-atmosphere interactions on Mars, Titan, and other worlds (including Earth) endure. But the animated conversations about potential innovations among the workshop participants suggest that at least some answers may lie just over the next dune crest.
Acknowledgments
S.D.’s contribution to this research was carried out at the Jet Propulsion Laboratory, California Institute of Technology, under a contract with NASA (80NM0018D0004). B.J.’s contribution to this research was carried out under a contract with NASA (80NSSC19K0542).
Author Information
Brian Jackson (bjackson@boisestate.edu), Boise State University, Idaho; Serina Diniega, NASA Jet Propulsion Laboratory, Pasadena, Calif.; Timothy Titus, U.S. Geological Survey, Flagstaff, Ariz.; Alejandro Soto, Southwest Research Institute, San Antonio, Texas; and Edgard Rivera-Valentin, Johns Hopkins University Applied Physics Laboratory, Laurel, Md.
Citation: Jackson, B., S. Diniega, T. Titus, A. Soto, and E. Rivera-Valentin (2023), The nitty-gritty forces that shape planetary surfaces, Eos, 104, https://doi.org/10.1029/2023EO230227. Published on 15 June 2023.
Text © 2023. The authors. CC BY-NC-ND 3.0
Except where otherwise noted, images are subject to copyright. Any reuse without express permission from the copyright owner is prohibited.
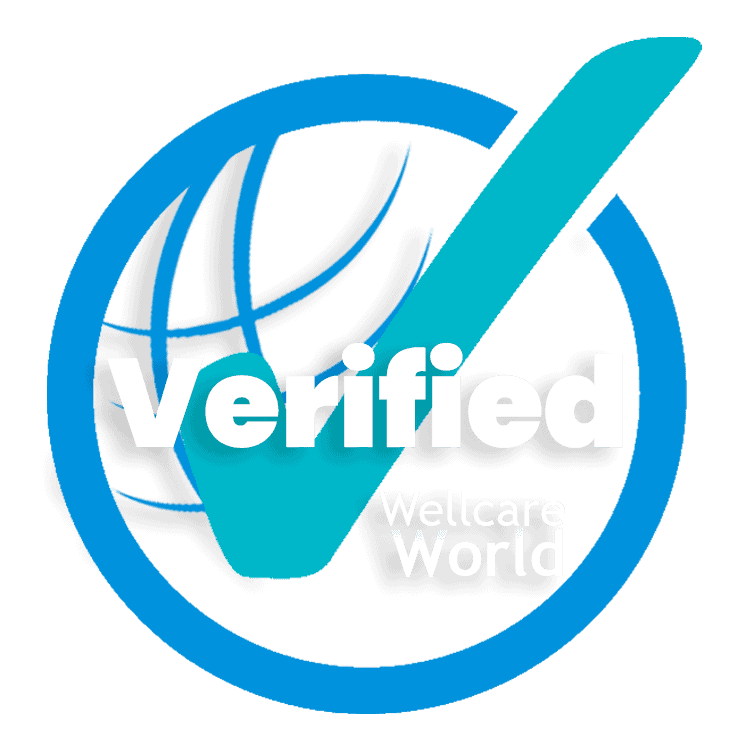
Offered by our Wellcare World friend at
Trending Also -> Physiotherapy Terahertz Technology TeraMD
Wellcare World specializes in providing the latest advancements in wellness technology, supplementation, and lifestyle changes that improve health and increase the quality of people's lives. To learn more, visit WellcareWorld.com and begin living a better life today.
Share Us With Others